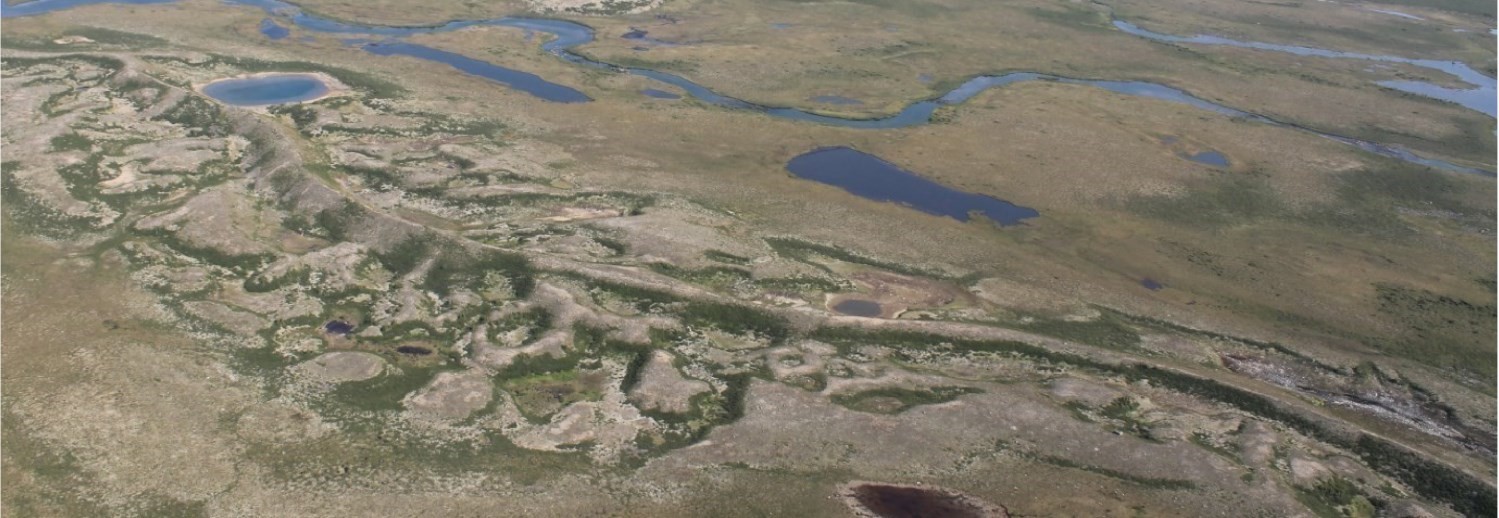
Last modification:
Etymology
The term esker was first used by Close (1867) in his review of Irish glacial geology, then introduced in North America by Upham (1876). The term, which comes from the Irish word eiscir (or Welsh escair) and means ridge, has long been used in the Anglo-Saxon world without distinction from the term kame (Levasseur, 1995), which has a different meaning nowadays. The Scandinavians prefer the terms ås or ôs and their plural derivatives osar or åsar. French geographers used the term os until the 1950s.
Description
The term esker is generally used to describe a thin straight or sinuous ridge in positive relief from the surrounding topography, which has been deposited by fluvioglacial processes. Within a single fluvioglacial corridor, numerous ridges can be seen, locally intersecting one another to form networks of anastomosing appearance.
Eskers are either continuous or discontinuous and are a few hundred metres to several hundred kilometres long, a few hundred metres wide and up to 200 m high (Wilson, 1939; Flint, 1971; Shilts et al., 1987; Warren and Ashley, 1994; Benn and Evans, 2010). The height and width are normally proportional to the length of the structure (Levasseur, 1995). The sides of ridges are generally steep, but may be less steep as a result of gravity processes, reworking, or due to certain inherent properties of the topography and ice substrate (Allard, 1974; Shreve, 1985a; 1985b). In some cases, they may be quite flat, such as those formed late in the deglaciation process.
Sediments found in an esker may consist of fluvioglacial silt, sand, gravel and boulders (Sugden and John, 1976; Warren and Ashley, 1994; Brennand, 2000; Benn and Evans, 2010), and are generally sorted and stratified. In general, the material is rounded to subrounded and indicates extensive meltwater transport. Eskers typically display normal sorting, while the core consists of coarser particles (pebbles and boulders) and the surface is made of stratified sands and gravels. Normal faults as well as crossbedding structures (Terwindt and Augustinus, 1985) could be observed in the sediment. They are respectively associated with glaciotectonic processes and episodic meltwater inflow.
Eskers may be isolated, but are most commonly arranged in swarms fed by a dendritic and complex network of subglacial channels (Flint, 1971). They are generally oriented subparallel to the ice flow direction (Tanner, 1937; Sugden and John, 1976) and located in areas of low relief (Flint, 1971; Lundqvist, 1979). The arrangement and spatial distribution of eskers generally reflect the retreat pattern of the ice margin, as they are generally arranged perpendicular to it.
Where valleys are more incised and the ice is thinner, the drainage pattern marked by eskers is arranged according to the relief (Mannerfelt, 1945), unless the ice flow direction is strongly perpendicular to these valleys (Flint 1971). The profile of the eskers is generally gently sloping down-ice, but some ridges can show moderate relief (Flint, 1971; Shreve, 1972).
Kettles typically border esker strings or observed within wider segments (Flint, 1971). Eskers occur in association with several other fluvioglacial bedforms, such as kames, proglacial moraines or proglacial or subglacial channels (Sugden and John, 1976). Frontal moraines are typically larger where esker strings meet.
Genesis
Few eskers could be observed at the time of their formation for obvious reasons of access, but the first reports of direct observations (Lewis, 1949) confirmed the previously existing theories (Hummel, 1874; De Geer, 1897; Mannerfelt, 1945; Flint, 1947).
Eskers may be deposited in a context of active ice (Synge, 1950; Barnett and Holdsworth, 1974; Shreve, 1972; 1985; Gorrell and Shaw, 1991) or decaying and relatively thin ice (Flint 1930; 1971; Shaw, 1972; Cheel, 1982; Hebrand and Åmark, 1989; Ashley et al., 1991). Sugden and John (1976) suggest that all eskers are preserved under inactive ice.
In the glacial environment, supraglacial, intraglacial or subglacial drainage systems may coexist (Röthlisberger, 1972; Sugden and John, 1976; Benn and Evans, 2010). These systems are constantly evolving, as water can move spatially within the glacier either by primary permeability (ice permeability) or secondary permeability (karst system, crevasse, moulin). For example, a supraglacial channel may transit to a subglacial tunnel via a moulin. The water comes from ice melting in the walls of these glacial tunnels. Subglacial channels, in which eskers are generated, have a tunnel shape and sit at the base of a glacier where meltwater is channelled under high pressure, overdeepening the overlying ice.
Eskers are tunnel fillings made of granular material that form sinuous ridges after the ice retreats (Benn and Evans, 2010). The high hydraulic pressure in subglacial tunnels ensures that they do not close under cryostatic pressure (Röthlisberger, 1972; Shreve, 1972; Sugden and John, 1976). This high hydrostatic pressure also allows for the transport of large quantities of sediment (Benn and Evans, 2010), which are deposited in the subglacial tunnel during a drop in hydraulic flow, typically following spring floods (Flint, 1971). This high pressure also explains the construction of esker segments that are not controlled by topography (Shreve, 1972; 1985; Sugden and John, 1976; Syverson et al., 1994; Benn and Evans, 2010).
The direction of esker flow is controlled by cryostatic pressure and hydraulic potential. Cryostatic pressure is controlled by the slope of the ice surface and the topography of the ice bed (Flint, 1971; Shreve, 1972). This explains why glacial drainage favours low-pressure areas, such as interlobar ice margin or ice margin confluence zone contexts (Veillette, 1986; Gustavson and Boothroyd, 1987; Warren and Ashley, 1994; Huddart et al., 1999). The direction of long esker trains is more representative of the late flow direction of a glacier than that measured from glacial bedforms such as drumlins (Embleton; 1964; Sugden and John, 1976).
At the ice margin, hydraulic pressure rapidly decreases and sediments are deposited, which results in subaerial or subaqueous outwash depending on the context of the margin, i.e. whether it is in contact with a proglacial water body (Banerjee and McDonald, 1975; Brennand, 2000). In the case of subaqueous outwash, several ice-proximal deltas could be recorded along an esker, reflecting the successive retreat of the glacial margin in contact with a body of water and producing eskers with a beaded appearance. Typically, the coarse fraction is deposited in the tunnel, while the finer fraction is deposited at the outlet of the tunnel as an outwash (Warren and Ashley, 1994). The subsequent retreat of the margin, and consequent removal of the granular source, will allow for the deposition of fine material onto the previously deposited esker ridge.
Usefulness
As well-defined sediment transport vectors, eskers are commonly used in glacioprospecting. Petrographic counts and identification of indicator minerals allow source rocks with economic potential for different metallogenic settings to be traced (Lee, 1965; McClenaghan and Kjarsgaard, 2007; Cummings et al., 2010).
In addition, eskers are a source of good quality, inexpensive aggregate used in the construction industry (Locat et al., 1990). Finally, eskers constitute a high-quality groundwater reserve for several Quebec municipalities and businesses. In fact, fine-grained deposits on the flanks of eskers are not very permeable and therefore conducive to the formation of aquifers (Nadeau, 2011).
Spatial Distribution
Eskers are ubiquitous in areas that have been glaciated (Storrar et al., 2013). Their numbers are greater in areas of rigid bedrock, for example in the Canadian Shield (Shilts et al., 1987; Clark and Walder, 1994), but are also found on other substrates (Benn and Evans, 2010). Current esker formation has been observed in Antarctica (Drews et al., 2017) and some similar forms have even been identified on Mars (Kargel, 1992).
In Quebec, eskers are listed in almost every regional work dealing with Quaternary geology, except in uninhabited areas affected by the existence of a postglacial lake or sea. In the Abitibi and James Bay Lowlands as well as the St. Lawrence Lowlands, eskers are generally buried, partially or entirely, under a layer of glaciomarine or glaciolacustrine clays or sands (Veillette et al., 2007; Nadeau, 2011; Lamarche and Dubé-Loubert, 2017).
References
Publications Available Through SIGÉOM Examine
LAMARCHE, O., DUBE-LOUBERT, H. 2018. GEOLOGIE DES DEPOTS DE SURFACE DE LA REGION DU LAC EVANS, EEYOU ISTCHEE BAIE-JAMES. MERN. RP 2017-02, 28 pages and 2 plans.
Other Publications
ALLARD, M., 1974. Géomorphologie des eskers abitibiens. Cahiers de géographie du Québec; volume 18, pages 271-296. doi.org/10.7202/021195ar
ASHLEY, G.M., BOOTHROYD, J.C., BORNS JR, H.W., 1991. Sedimentology of late Pleistocene (Laurentide) deglacial-phase deposits, eastern Maine; An example of a temperate marine grounded ice-sheet margin. Geological Society of America; Special Paper 261, pages107-125. doi.org/10.1130/SPE261-p107
BANERJEE, I., MCDONALD, B.C., 1975. Nature of esker sedimentation. In JOPLING, A.V., MCDONALD, B.C. (Eds), Glaciofluvial and glaciolacustrine sedimentation. Society of Paleontologists and Mineralogists; Special Publication 23, pages 132-154. doi.org/10.2110/pec.75.23.0132
BARNETT, D. M., HOLDSWORTH, G., 1974. Origin, morphology, and chronology of sublacustrine moraines, Generator Lake, Baffin Island, Northwest Territories, Canada. Canadian Journal of Earth Sciences; volume 11, pages 380-408. doi.org/10.1139/e74-036
BENN, D.I., EVANS, D.J.A., 2010. Glaciers and glaciations, second edition. Routledge, Taylor & Francis Group, London and New York, 802 pages. doi.org/10.4324/9780203785010
BRENNAND, T.A., 2000. Deglacial meltwater drainage and glaciodynamics: inferences from Laurentide eskers, Canada. Geomorphology; volume 32, pages 263-293. doi.org/10.1016/S0169-555X(99)00100-2
CHEEL, R.J., 1982. The depositional history of an esker near Ottawa, Canada. Canadian Journal of Earth Sciences; volume 19, pages 1417-1427. doi.org/10.1139/e82-123
CLARK, P.U., WALDER, J.S., 1994. Subglacial drainage, eskers, and deforming beds beneath the Laurentide and Eurasian ice sheets. Geological Society of America Bulletin; volume 106, pages 304-314. doi.org/10.1130/0016-7606(1994)106<0304:SDEADB>2.3.CO;2
CLOSE, M.H., 1867. Notes on the general glaciation of Ireland. Journal of the Royal Geological Society of Ireland; volume 1, pages 207-242.
CUMMINGS, D.I., RUSSELL, H.A.J., SHARPE, D.R., KJARSGAARD, B.A., 2010. Eskers as Mineral Exploration Tools: An Annotated Bibliography. Geological Survey of Canada; Open File 6560, 102 pages. doi.org/10.4095/286002
DE GEER, G.J., 1897. Om rullstensåsarnas bildningssatt. GeologiskaFöreningensi Stockholm Förhandlingar; Bd. 19, pages 366-388. doi.org/10.1080/11035899709448614
DREWRY, D., 1986. Glacial geologic processes. Edward Arnold, Baltimore, 700 pages.
DREWS, R. PATTYN, F., HEWITT, I.J., NG, F.S.L., BERGER, S., MATSUOKA, K., HELM, V., BERGEOT, N., FAVIER, L., NECKEL, N., 2017. Actively evolving subglacial conduits and eskers initiate ice shelf channels at an Antarctic grounding line. Nature Communications; volume 8, 10 pages. doi.org/10.1038/ncomms15228
EMBLETON, C., 1964. Sub-glacial drainage and supposed ice-dammed lakes in North-East Wales. Proceedings of the Geologists’ Association; volume 75, pages 31-32. doi.org/10.1016/S0016-7878(64)80009-2
FLINT, R.F., 1930. The origin of the irish »Eskers ». Geographical Review; volume 20, pages 615-630. doi.org/10.2307/209015
FLINT, R.F., 1947. Glacial geology and the Pleistocene epoch. New York, John Wiley & Sons, pages 150-155.
FLINT, R.F., 1971. Glacial and Quaternary geology. New York, John Wiley & Sons, 892 pages.
GORRELL, G., SHAW, J., 1991. Deposition in an esker, bead and fan complex, Lanark, Ontario, Canada. SedimentaryGeology; volume 72, pages 285-314. doi.org/10.1016/0037-0738(91)90016-7
GOULET, C., 2010. La prospection glacio-sédimentaire en zone d’écoulements glaciaires multiples comme outil pour l’exploration de gisements aurifères: le cas du secteur de Meadowbank au nord de Baker Lake, Nunavut. Université du Québec à Montréal, master’s thesis, 107 pages. Source
GUSTAVSON, T.C., BOOTHROYD, J.C., 1987. A depositional model for outwash, sediment sources, and hydrologic characteristics, Malaspina Glacier, Alaska: A modern analog of the southeastern margin of the Laurentide Ice Sheet. Geological Society of America Bulletin; volume 99, pages 187-200. doi.org/10.1130/0016-7606(1987)99<187:ADMFOS>2.0.CO;2
HEBRAND, M., ÅMARK, M., 1989. Esker formation and glacier dynamics in eastern Skane and adjacent areas, southern Sweden. Boreas; volume 18, pages 67-81. doi.org/10.1111/j.1502-3885.1989.tb00372.x
HUDDART, D., BENNETT, M.R., GLASSER, N.F., 1999. Morphology and sedimentology of a high-arctic esker system: Vegbreen, Svalbard. Boreas; volume 28, pages 253-273. doi.org/10.1111/j.1502-3885.1999.tb00219.x
HUMMEL, D., 1874. Om rullstenbildningar. K. Svenska Vetenskaps-Akademiens Förhandlingar, Bihang til; volume 2, 36 pages.
KARGEL, J.S., STROM, R.G., 1992. Ancient glaciation on Mars. Geology; volume 20, pages 3-7. doi.org/10.1130/0091-7613(1992)020<0003:AGOM>2.3.CO;2
LEE, H.A., 1965. Investigation of eskers for mineral exploration. Geological Survey of Canada; Paper 65-14, pages 1-17. doi.org/10.4095/100988
LEVASSEUR, D., 1995. Les eskers : essai de synthèse bibliographique. Géographie physique et Quaternaire; volume 49, pages 459-479. doi.org/10.7202/033066ar
LEWIS, W.V., 1949. An esker in process of formation: Böverbreen, Jotunheimen, 1947. Journal of Glaciology; volume 1, pages 314-319. doi.org/10.3189/S0022143000010066
LOCAT, J., CHAGNON, J.-Y., BRAZEAU, A., BÉRUBÉ, M.-A., BÉLANGER, J., 1990. L’inventaire des granulats au Québec. Bulletin de l’Association Internationale de Géologie de l’Ingénieur, volume 41, pages 107-116. doi.org/10.1007/BF02590211
LUNDQVIST, J., 1979. Morphogenetic Classification of Glacifluvial Deposits. Sverigesgeologiska undersökning: Serie C, Avhandlingar och uppsatser; volume 767. 72 pages.
MANNERFELT, C.M.S., 1945. Några Glacialmorfologiska Formelement: Och Deras Vittnesbörd Om Inlandsisens Avsmält-Ningsmekanik I Svensk Och Norsk Fjällterräng. Geografiskaannaler; volume 27, 239 pages. doi.org/10.1080/20014422.1945.11880732
MCCLENAGHAN, M.B., KJARSGAARD, B.A., 2007. Indicator Mineral and Surficial Geochemical Exploration methods for Kimberlite in Glaciated Terrain; Examples from Canada. In Goodfellow, W.D. (ed), Mineral Deposits of Canada: A Synthesis of Major Deposit-Types, District Metallogeny, the Evolution of Geological Provinces, and Exploration Methods, Geological Association of Canada, Special publication No.5, pages 983-1006. Source
NADEAU, S., 2011. Estimation de la ressource granulaire et du potentiel aquifère des eskers de l’Abitibi-Témiscamingue et du sud de la Baie-James (Québec). Université du Québec à Montréal; mémoire de maîtrise, 34 pages. Source
RÖTHLISBERGER, H., 1972. Water Pressure in Subglacial Channels. Journal of Glaciology; volume 11, pages 177-203. doi.org/10.3189/S0022143000022188
SHARPE, D.R., 1988. Glaciomarine fan deposition in the Champlain Sea. In GADD, N.R. (Ed), The Late Quaternary development of the Champlain Sea Basin. Geological Association of Canada; Special paper 35, pages 63-82.
SHAW, J., 1972. Sedimentation in the ice‐contact environment, with examples from Shropshire (England). Sedimentology; volume 18, pages 23-62. doi.org/10.1111/j.1365-3091.1972.tb00002.x
SHILTS, W.W., AYLSWORTH, J.M., KASZYCKI, C.A., KLASSEN, R.A., 1987. Canadian Shield. In GRAF, W.L. (ed), Geomorphic systems of North America: Boulder Colorado, Centennial Special Volume 2, Geological Society of America, pages 119-161. doi.org/10.1130/DNAG-CENT-v2.119
SHREVE, R.L., 1972. Movement of water in glaciers. Journal of Glaciology; volume 11, pages 205-214. doi.org/10.3189/S002214300002219X
SHREVE, R.L., 1985a. Esker characteristics in terms of glacier physics, Katahdin esker system, Maine. Geological Society of America Bulletin; volume 96, pages 639-646. doi.org/10.1130/0016-7606(1985)96<639:ECITOG>2.0.CO;2
SHREVE, R.L., 1985b. Late Wisconsin ice-surface profile calculated from esker paths and types, Katahdin esker system, Maine. Quaternary Research; volume 23, pages 27-37. doi.org/10.1016/0033-5894(85)90069-9
STORRAR, R.D., STOKES, C.R., EVANS, D.J.A., 2013. A map of large Canadian eskers from Landsat satellite imagery. Journal of Maps; volume 9, pages 456-473. doi.org/10.1080/17445647.2013.815591
SUGDEN, D.E., JOHN, B.S., 1976. Glaciers and landscape, a geomorphological approach. John Wiley and Sons, New York, 376 pages.
SYNGE, F.M., 1950. The glacial deposits around Trim, Co. Meath. Proceedings of the Royal Irish Academy. Section B: Biological, Geological, and Chemical Science; volume 53, pages 99-110. Source
SYVERSON, K.M., GAFFIELD, S.J., MICKELSON, D. M., 1994. Comparison of esker morphology and sedimentology with former ice-surface topography, Burroughs Glacier, Alaska. Geological Society of America Bulletin; volume 106, pages 1130-1142. doi.org/10.1130/0016-7606(1994)106<1130:COEMAS>2.3.CO;2
TANNER, V., 1937. The problems with the eskers; V – The Tälisvuom’puoltsha esker in Enontekis, Lapmark. Fennia; volume 63, 31 pages.
TERWINDT, J.H.J., AUGUSTINUS, P.G.E.F., 1985. Lateral and longitudinal successions in sedimentary structures in the Middle Mause esker, Scotland. Sedimentary Geology; volume 45, pages 161-188. doi.org/10.1016/0037-0738(85)90001-6
UPHAM, W., 1876. On the origin of kames or eskers in New Hampshire. Proceedings of the American Association for the Advancement of Science; volume 25, pages 216-225.
VEILLETTE, J.J., 1986. Former southwesterly ice flows in the Abitibi-Timiskaming region: implications for the configuration of the late Wisconsinan ice sheet. Canadian Journal of Earth Sciences; volume 23, pages 1724-1741. doi.org/10.1139/e86-159
VEILLETTE, J.J., CLOUTIER, V., DE CORTA, H., GAGNON, F., ROY, M., DOUMA, M., BOIS, D., 2007. A complex recharge network, the Barraute esker, Abitibi, Quebec. 60th Canadian Geotechnical Conference and 8th Joint CGS/IAH-CNC Groundwater Conference, October, pages 347-354.
WALDER, J.S., 1982. Stability of sheet flow of water beneath temperate glaciers and implications for glacier surging. Journal of Glaciology; volume 28, pages 273-293. doi.org/10.3189/S0022143000011631
WALDER, J.S., 2010. Röthlisberger channel theory : its origins and consequences. Journal of Glaciology; volume 56, pages 1079-1086. doi.org/10.3189/002214311796406031
WARREN, W.P., ASHLEY, G.M., 1994. Origins of the ice-contact stratified ridges (eskers) of Ireland. Journal of Sedimentary Research; volume A64, pages 433-449. doi.org/10.1306/D4267DD9-2B26-11D7-8648000102C1865D
WEERTMAN, J., 1972. General Theory of Water Flow at the Base of a Glacier of Ice Sheet. Reviews of Geophysics and Space Physics; volume 10, pages 287-333. doi.org/10.1029/RG010i001p00287
WILSON, J.T., 1939. Eskers of north-east of Great Slave Lake. Transactions of the Royal Society of Canada; section IV, volume 33, pages 119-129.
Contributors
First publication |
Olivier Lamarche, P. Geo., M.Sc. olivier.lamarche@mern.gouv.qc.ca, Hugo Raymond, GIT, B.Sc. (redaction); Hugo Dubé-Loubert, P. Geo., Ph. D. (critical review); François Leclerc, P. Geo., Ph.D. (template and content compliance); Simon Auclair, P. Geo., M.Sc. (editing); Céline Dupuis, P. Geo., Ph.D. (English version); André Tremblay (HTML editing). |